Backward Inhibition: Evidence and Possible Mechanisms
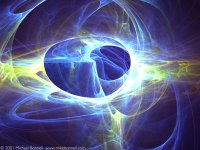
Some evidence for the idea that task sets must be inhibited comes from Mayr & Keele's 2000 JEP:G article, in which they conduct a series of experiments where subjects select the object "that doesn't belong" among a set of 4 objects. Three objects are the same color, while one is a different color. Another object has a different orientation than the other three. And a third of the four displayed object is moving, while the others remain still. How, then, does a subject decide which one doesn't belong?
Mayr and Keele present a "task cue" a certain interval (the "CSI") before they display these objects - this task cue either indicates whether the oddball judgment should be made on the basis of color, orientation, or motion. After responding, the subjects are not presented with another cue for a certain interval (the response-cue interval, or RCI). By varying the CSI and the RCI as follows, certain patterns might emerge:
1) Short RCI & Short CSI: in this situation, subjects should have little time to prepare a new task-set representation, and the previous task-set should have had little time to decay. Therefore, subjects should have more difficulty switching back to a task-set that had been used two trials ago - in other words, one that had recently been used, but then abandoned - than switching to a task set that had been used more than two trials ago. (This difference is referred to as "backward inhibition," or BI.)
2) Long RCI & Short CSI: here, the old task-set will have decayed, but subjects should have had little time to instantiate a new task set. In this case, subjects should show reduced backward inhibition (compared to the situation in #1) when switching to a cue that had been recently used but then subsequently abandoned.
3) Short RCI & Long CSI: in this case, subjects have a long time to instantiate a new task set, they might be more able to activate the previously-used-but-more-recently-abandoned task set, and thus show less backward inhibition than in 1 (and possibly also than in 2).
Although 2 & 3 did show less BI than 1, there was no difference between the backward inhibition found in 2) and 3) above. This indicates that switching to a previously abandoned task set is not made easier by having longer to prepare - suggesting that "task set inertia" appears to be unrelated to how strongly one is able to activate the new task. Mayr and Keele interpret this as evidence that switching involves an inhibitory process acting upon previous task sets.
These results suggest that "backward inhibition" is not actually a side-effect of not having fully activated the now-relevant task set, which would be an explanation easily backed-up by many computational models of task-switching. Instead, it suggests that something more complicated is going on - perhaps old task-sets are actually inhibited. But does this happen as a result of lateral inhibition among competing task-sets, or is it an active top-down process of executive control?
In a third experiment, the authors attempt to dissociate these two possible forms of inhibition ("bottom-up" versus "top-down" executive control) by changing the stimulus display such that one object differed from other three in terms of size, while another object differed from the others in terms of another dimension (color, motion or orientation). However, subjects were told only that size was always irrelevant, and that they should pick the "oddball" item on the basis of its features. In another condition, size was still irrelevant, but subjects were told which dimension would differentiate the oddball from the other items (e.g., they might be told "color").
If backward inhibition is actually a top-down phenomenon, one would expect to see BI effects only in the case where subjects were told which dimension would be relevant, because they would have deliberately suppressed the older task set. In contrast, those in the "bottom-up" condition (where they were not told the relevant dimension) should not show BI if it inhibition actually a control mechanism engaged by top-down mechanisms.
Essentially, this is what the authors found. In further experiments, they demonstrate that backwards inhibition may make up the bulk of what is called "residual shift cost" - the amount of time taken by participants to switch to a task despite having been given ample time to prepare for the new task.
What mechanisms might subserve this process? It seems hard to imagine that the brain is actively suppressing previously used but currently irrelevant items. A mechanism that mimics these effects through active maintenance and lateral inhibition along would be far more parsimonious.
One possibility is that recently used task sets are never completely abandoned, but instead enjoy gradually diminishing activation as new task sets are activated. If one assumes that task sets are affected by processing both at the executive level and at the stimulus level, which seems likely (and is assumed by Mayr & Keele), then an attractor network can be viewed as a candidate model.
In this case, switching to a new task set through top-down control would involve biasing the attractor state of the previous task towards the current task. In this case, traces of the previous task representation may become incorporated into the new stable attractor state, which represents the current task. However, after a second task-switch, it may take longer for the attractor to "revert" to a previous state. This might occur because the attractor basin - the diversity of representations that lead to the same response - had been widened to accomodate traces of the previous task. In contrast, switching to a less recently performed task might be paradoxically easier, since it involves moving to a substantially different attractor state, far outside the basin of attraction in the last 2 most recently performed tasks.
Of course, these possible mechanistic explanations are completely speculative; this account would obviously be strengthened by an actual implementation of the backward inhibition phenomenon within an attractor network that lacks directed inhibition. Yet other directed inhibition phenomena would be difficult to explain in this framework, such as evidence reviewed yesterday.
On the other hand, if directed inhibition is truly at work in the brain, one needs to explain the relative lack of long-range inhibitory connections in cortex. Thus, we arrive at a conundrum: behavioral research appears to show directed inhibition at work, but neurobiology does not provide an easy way of explaining the mechanism. It seems safe to claim that biologically-plausible computational models will be important in resolving this apparent discrepancy.
1 Comments:
Hi, I came across this blog page after a google scour! just wondering who the author of this blog post is? I am doing my PhD on Backward Inhibition with Dr. George Houghton. It would be good to chat with the author via email to discuss any ongoing work in the suggestions proposed in the blog. My email is:
psp48d@bangor.ac.uk.
Cheers.
Post a Comment
<< Home