fMRI of the Stop Signal Task: What computations support "stopping"?
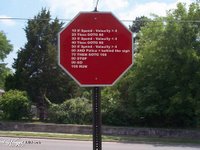
Authors Aron & Poldrack argue that rIFC may target a region of the basal ganglia known as subthalamic nucleus (STN). The STN is a special region of basal ganglia in that it is thought to excite the internal/medial globus pallidus as well as the substantia nigra pars reticulata, both of which then inhibit the thalamus. Thus, the end result of rIFG activation may be a general "clamping down" of thalamic output, if the rIFG does indeed target the STN - this is known as the "hyperdirect pathway."
It is not controversial that STN accomplishes such inhibition both neurally and behaviorally. Aron & Poldrack review evidence showing that STN stimulation improves stop signal reaction time (thought to be a measure of how much time is required for subjects to "cancel" a motor action). Likewise, STN damage decreases stop signal reaction time.
What is controversial, however, is the existence of the "hyperdirect pathway" whereby rIFG directly activates STN and thus inhibits cortical activity. The more commonly accepted mechanism is known as the "indirect pathway," in which cortical areas activate striatal pathways, which themselves disinhibit STN, by inhibiting the external globus pallidus (you can probably see where it gets its name!).
To identify which of these two possible neural networks are active in motor inhibition, the authors put 18 healthy, right-handed adults into an fMRI scanner, where they were presented with a screen containing either a left- or right-pointing arrow. Each subject was told to press a corresponding left or right key, unless a sound was played after the presentation of the arrow, in which case subjects had to refrain from pressing the button (although this sound was only played on 25% of trials). The delay between the display of the arrow and the onset of the sound was dynamically calibrated to each subject while in the scanner, such that each subject was getting around 50% of the "stop trials" correct and 50% incorrect. The value of this delay is known as the stop signal delay, or SSD. This is then subtracted from the median reaction time on go trials to arrive at the stop signal reaction time, or SSRT.
The classic interpretation of SSRT is that it represents the rate of diffusion of a "stopping process," whereas the reaction time on normal go trials represents the rate of diffusion of a "go process." This is known as Logan's race model. The behavioral results were largely in line with this conceptual model, and showed that SSRT and RT on go trials were uncorrelated, supporting the "race model's" assumption that processes involved in "stop" and "go" trials are distinct. Secondly, the median reaction time for incorrect stop trials (i.e., where subjects incorrectly made a response) was faster than the median reaction time on correct go trials, which is again consistent with the race model. SSRTs were within the normal range of around 120 ms.
The fMRI results showed that all "stop" trials were associated with activity in right inferior frontal cortex and STN, consistent with the hyperdirect account. However, failed "stop" trials demonstrated strongly decreased motor cortex activity (M1) towards the end of each trial relative to both Go trials and correct "stop" trials. This was interpreted to indicate that inhibition was "triggered at the neural level, even if it was ineffective at the behavioral one." Conversely, correctly inhibited "stop" trials were associated with increased putamen activity relative to incorrect stop trials, which Aron & Poldrack persuasively argue to reflect higher conflict, resulting from a slower "go" process on those trials.
In support of the "hyperdirect" pathway, activity in rIFC was correlated with activity in STN , and activity in both regions was stronger for subjects with faster SSRTs. Other analyses suggest that while the degree to which rIFC is recruited does not depend on SSD, activity in STN, pSMA and globus pallidus does, such that those regions are more active the longer the SSD (and thus the closer to execution the response is).
There are several complications in interpreting these results. First, the decreased M1 activity on failed stop trials may have to do with the length of those trials as compared to the length of correct Go trials, which showed a very similar but protracted temporal profile. Furthermore, if signal strength reductions are to indicate inhibition, it is strange that "go" trials would show this pattern - because clearly inhibition is neither needed nor performed on correct "go" trials! This suggests that inhibition may not be an accurate term for what is observed in this case.
Secondly, there are at least three reasons that STN, pSMA and globus pallidus might be more active on trials in which the stop signal occurs later relative to trials where it occurs earlier. The possibility endorsed by Aron & Poldrack is that in late-signal trials motoric inhibition must be performed (since the movement may already be initiated), whereas in early-signal trials more "cognitive" inhibition must be performed (since only movement planning will have begun). However, it's also possible this is an an artifact of the longer "go" process which is necessarily present on these trials, as was argued to be the case for putamen activity. Or it could be that this activity is akin to the "lateralized readiness potential" detected by EEG studies of stop signal, in which there is increasingly negative frontal activity as a stop signal trial wears on. Either way, the conclusion that this reflects greater motoric inhibition is simply premature.
Third, if rIFC actually executes inhibition, it is strange that activity in that region is insensitive to the type of inhibition that would have to be performed. For example, one would expect different patterns of activity in rIFC for trials requiring motoric inhibition relative to those requiring "cognitive inhibition," and yet this was not observed. Instead, the activity in this region is more compatible with an account where rIFC is actively monitoring task cues/performance and orchestrating subsequent control or selection processes, rather than accomplishing inhibition per se.
This perspective is also compatible with a wide variety of findings on the functional role of rIFC. For example, right inferior frontal activity has been associated with both deviance and novelty detection in an oddball paradigm. Detection of unusual stimuli would be important for a region involved in "task monitoring." Likewise, rIFC is more activated by negative than positive feedback. Finally, rIFC is also most active during conditions of high WM load, also suggesting a role for that region in selection or monitoring processes, but not necessarily inhibition.
Related Posts:
Noninhibitory Accounts of Negative Priming
Inhibition and rTMS
Backward Inhibition: Evidence and Possible Mechanisms
Inhibition from Excitation: Reconciling Directed Inhibition with Cortical Structure
The Tyranny of Inhibition
1 Comments:
Excellent Post!
Post a Comment
<< Home